Surely you've heard of the butterfly effect: The energy produced by a butterfly beating its wings near the sea on one side of the world generates a tidal wave thousands of miles away. In an interconnected, global economy, the disruption of supply chains by those tidal waves, tsunamis, banking crisis, and volcanoes are all too real.
Since disruption is a given, the real measure of an organization isn't how it plans to manage risk, but how the resilient company bounces back when something happens.
In The Power Of Resilience: How The Best Companies Manage The Unexpected, Yossi Shefi shows that supply chain risk management “is a balancing act between taking on the risks involved in new products, new markets, and new processes - all crucial for growth - and the resilience created by advanced risk management.”
Chapter 1. A Quake Breaks A Supply Chain is printed below. You can read a Q&A with Sheffi about the new book on Supply Chain 24/7 (courtesy of Supply Chain Management Review).
Afternoon, March 11, 2011, Japan
Jackie Sturm and Jeff Selvala sat with a dozen other Intel colleagues in the Narita Airport outside Tokyo, Japan. They were waiting for the long flight home after a conference with their Japanese suppliers. At the conference, they had discussed routine operations, quality improvements, forthcoming production plans, and technology roadmaps to ensure a continuing smooth supply of the hundreds of arcane materials that feed Intel's $36.5 billion in fabrication facilities (fabs). Staying ahead of the world's ravenous demand for chips required choreographing a global supply chain, which meant traveling to Japan to visit the many silicon wafer and specialty chemical suppliers who produce the ultrapure materials needed for Intel's chips.
Change of Plans
Unbeknownst to the Intel employees - and the world - a horrendous accumulation of geophysical energy had reached a breaking point. At 2:46 pm local time, some 72 kilometers off the coast of Japan, the Pacific plate broke its locked fault line and began to shear downward and westward while the Okhotsk plate beneath northern Japan thrust upward and eastward. More than 1,000 years of accumulated strain broke free, sending a seismic shockwave racing at over 7,000 kilometers per hour through the solid rock of the floor of the Pacific. The shock caused more of the surrounding fault lines to rip like a broken zipper. A 500-kilometer-wide sheet of the earth's plate east of Japan slid under the islands, the coastline sank about one-half meter, the adjacent sea- bed rose up to three meters, and that part of Japan lurched over two meters toward North America. As the sheet shifted, a prolonged and growing shockwave radiated in all directions. In less than a minute, the first earthly shudders reached Japan.
A few minutes later, the shockwave reached the Narita airport. Those who live in Japan or spend any amount of time there know about the ordinary tremors that remind the Japanese of where they live. But this was no ordinary tremor. In Narita, the quake began with a long low rumble that turned into a rolling motion that steadily gathered strength. This was a big one and everyone knew it. As the quake built, people who were standing sat down. As the strength increased, many sat on the floor. And there they remained for four to five minutes as the quake rumbled on.
The Japanese know they live on a treacherous island prone to quakes, tsunamis, and even the occasional volcano. Born of bitter experience and untold hundreds of thousands of deaths throughout their history, today's Japanese erect heavily reinforced structures and heady seawalls to survive most of the worst that the earth can throw at them. But history does not always repeat itself, and the disaster that followed was worse than anything the Japanese planners had anticipated.
The Living Assess the Situation
When the shaking stopped at Narita, everyone looked around and breathed a sigh of relief. They were alive and unhurt. The airport's well-designed buildings had withstood the shaking. Then came the fears: worries about the safety of family, friends, and coworkers in Japan. Intel has some 600 employees in Japan at two facilities, plus innumerable friends and acquaintances at the company's long-term suppliers. The travelers also worried about what their families would think when they heard of the devastating quake. Immediately, people started calling, texting, and emailing to determine if everyone was safe and to tell everyone that they were safe.
The Intel employees and others found communications to be difficult in the immediate aftermath. The barrage of anxious callers overwhelmed local cell towers. Internet access was sporadic. Long lines of people clustered around working landline phones. Moreover, no calls were reaching the most damaged areas. The disaster had knocked out power plants, downed power lines, and severed telecommunications cables. Three prefectures had no power, and two additional ones had partial blackouts.
The Quake Was the Least of the Worries
Although the worst of the shaking had stopped and inspectors were rushing around Narita to confirm the lack of damage, the disaster was only just beginning. Earthquakes on the Pacific's ring of fire have the ironic tendency to produce walls of water. When that Pacific plate of rock dropped, the Japanese land mass lifted, as did the thousands of meters of water above the seabed. Drop a stone in a bathtub and the ripples expand to slosh against the sides. Move thousands of square kilometers of rock in an underwater quake, and the sloshing is immense. The Japanese know this, too, and they have elaborate processes for detecting quakes, estimating the potential magnitude of any tsunami spawned by a quake, and alerting seaside authorities of potential wave heights.
As Japan's network of 3,700 seismic sensors relayed data on the quake, the seismologists of the Japan Meteorological Agency (JMA) quickly estimated the size of the quake and tsunami and sounded the alarm in the first three minutes of the event. Unfortunately, the system was actually too quick - it used only the first minute of quake data, not the full duration of the shaking, in initially estimating a 7.9 magnitude value to the quake. In reality, the quake was a magnitude 9, making it the largest earthquake ever to hit Japan in the country's 1,500 years of recorded history. Solid engineering, reinforced concrete, and lofty seawalls can handle a lot, but a magnitude 9 quake is more than a lot. An earthquake of magnitude 9 on the moment magnitude scale releases over 31.6 times more destructive energy than a magnitude 8 and 44.7 times more energy than the initially estimated 7.9.
About half an hour after the shaking ended, a tsunami two to three times higher than projected overwhelmed coastal defenses and inundated the shores nearest to the quake's epicenter. In some areas, a fateful conspiracy of the direction of the tsunami and the shapes of land, harbors, and sea bottom acted to concentrate the tsunami into a 20-meter-high wall of water. Large container ships were lifted over piers and wharves and were shoved inland as the water invaded the land. Along the Sendai coast, seawater flowed more than four kilometers inland across the broad coastal plain. As the waters retreated, houses, buildings, cars, and thousands of people were dragged out to sea. In some areas, rubble created by the quake was simply wiped from the land.
The violent ground motion, waves, and fires started by the quake damaged or destroyed some 1.2 million buildings across a large swath of northern Japan. Far worse was the human toll. Over 19,000 people lost their lives, some 50,000 were injured, and 400,000 became homeless. Of those who died, nearly 3,000 were never found - sucked into briny depths. The tsunami was responsible for 80 percent of the destroyed buildings and over 94 percent of the deaths.
Too Hot to Handle
Even as the Japanese quickly started their rescue and inspection efforts, more phases of the disaster began unfolding. Some 180 kilometers away from the quake's epicenter lay the nuclear power complex of Fukushima Daiichi, with six nuclear reactors. The reactor complex was part of Japan's strategy of avoiding the risks of reliance on foreign supplies of fossil fuels. On March 11, three reactors were live and three were shut down for routine maintenance. When the quake struck, the three operating reactors immediately performed an emergency shutdown, dropping control rods into the reactor to suspend power production.
The Japanese thought they understood the seismic risks of Fukushima. A long historical record of earthquakes and careful modeling of the faults near Fukushima suggested that the region had manageable seismic and tsunami risks. “With firm geological foundations and major earthquakes rare, Fukushima is a safe and secure place to do business,” said the government website for Fukushima prefecture.
But the seismic modeling failed to include the potential for co- seismic coupling - a knock-on effect in which the rupture in one fault induces larger ruptures in the many other faults around the Japanese islands. The violence of the quake exceeded the design specifications of three of the reactors; eyewitness accounts and postquake activities suggest significant quake-related damage to the reactor cooling systems.
The cold seawater that was the reason for the plant's seaside location became the facility's ultimate nemesis. About 50 minutes after the quake, the tsunami arrived. The sea rose to the 10-meter-high level of the plant, began flooding it, and kept rising. High waters soaked various parts of the plant for between 30 minutes to one hour. Seawater flooded the backup diesel generators, thoroughly soaked critical controls with corrosive seawater, and shorted key electrical systems.
Even when powered down, the nuclear byproducts inside the reactor core's fuel rods generated tremendous heat. Without a continuous supply of cooling water, the remaining water sitting in the reactor chamber began to boil and raise the pressure of the chamber. To prevent catastrophic failure, emergency valves vented radioactive steam. Worse, as the water boiled and exposed the core, high-temperature chemical reactions produced explosive hydrogen gas.
If too much of the core is exposed, temperatures can rise to 2,800°C, melt the core assembly, and potentially allow uncontrolled fission to create vastly larger amounts of heat and radioactive debris. The ultra-hot molten mass of reacting fuel could literally melt down through the bottom of the reactor, through any other containment walls, and begin melting into the concrete foundation of the reactor.
Nor were the reactors the only concern. The Fukushima operators faced a similar threat with the spent fuel rods sitting in the adjacent cooling pools. Without recirculating water, these pools began to heat toward the boiling point. If the pools boiled and evaporated, the rods would be exposed, become extremely hot, and release radioactive materials directly into the air.
The quake and tsunami led to a desperate battle to cool the reactors and fuel pools. At every turn, the workers faced horrible choices with significant risks of radiation leakage, explosions, and irreversible damage to the reactors' systems. The plant's lack of power and damaged sensor infrastructure meant the operators had limited visibility into conditions inside the reactor building and had limited control.
The workers at the Fukushima tried valiantly to cool the reactor cores and the fuel pools. They brought in fire trucks and helicopters to dump water into the stricken reactor buildings. They sent rotating crews of workers and firemen to limit each worker's exposure to radiation. Rather than risk an immediate explosion because of lack of water, they used seawater in an attempt to cool the reactor, knowing that the salt could utterly ruin the reactor and potentially clog the pipes.
Yet without power, without large supplies of fresh water, without full sensor data on what was happening, and without the ability to enter the extremely radioactive reactor buildings, the workers had little hope of preventing a catastrophe. Only 24 hours after the tsunami, a hydrogen explosion shattered the reactor unit #1 building, followed by similar explosions in unit #3 (March 14), unit #2 (March 15), and unit #4 (March 15). Each explosion brought renewed fears of major radiation releases and created unknown amounts of damage to internal systems. Post-analysis of the disaster showed that unit #1 suffered a complete meltdown, and units #2 and #3 suffered partial meltdowns.
From Reactors to Reactions
The third phase of the disaster arose from the horrible realities and uncertainties about radiation spewing from the stricken reactors. On many days, the radiation drifted out to sea to rain down into the Pacific. But on other days, radiation moved inland, motivating evacuations of the area around the reactors and causing concerns for the water supplies of Japanese cities. (Detectable amounts of radioactive materials even crossed the Pacific to appear in US air and rain samples.) The invisible nature of radiation and the potential that it might coat objects or be absorbed into plants and animals created frightening uncertainties about the short-term and long-term safety of residents, businesses, farmers, and fishermen in the affected area. It also created worries for Japanese companies with parts and goods in the area. No one knew how far the radiation might spread and whether it could contaminate shipments sent to customers around the world.
TV images showed the grim aftermath of the explosions and fires - shattered buildings and a miasma of radioactive smoke rising unabated from the remains. News stories buzzed with data on rising heat levels, falling coolant levels, new attempts to stabilize the situation, and new fears of worsening conditions. As the threat of radiation grew, Japanese authorities created progressively larger and larger evacuation zones at 2, 10, 20, and 30 kilometers and asked citizens over a larger area to remain indoors. But were the evacuation zones large enough? Revelations showed that the plant's operator (TEPCO) and the Japanese government had repeatedly downplayed the extent of the crisis.
Uncertainties about the amount of radiation spewing from the smoldering plant and the potential shifting direction of the winds added to the anxiety. Intel considered evacuating all of its Japanese personnel, but the personnel themselves vetoed the idea.
Companies with business in Japan, who bought Japanese products, or who simply moved goods through Japanese waters began to wonder: were their goods radioactive? Fear of radioactive foods, materials, and dust created questions about the safety of Japanese exports. Intel and others worried about freight transiting Japanese ports - radioactive dust might settle on or get into shipping containers passing through the area. This potentially affected 7,000 of Intel's shipping lanes, because it affected lanes with Japanese origins or destinations as well as all Asia-Pacific lanes that might sail near Japanese waters.
Another company, chemical giant BASF, worried about radioactive ingredients getting into consumer products. For example, BASF supplies the ingredients that go into lipstick manufactured and sold by Procter & Gamble. To assure itself and its customers of the safety of the ingredients originating from Japan, BASF installed Geiger counters on both the Japanese and German sides of its supply chain.
Intel had an added worry. Even if the chips themselves were clean of any radioactive contamination, exposure to radiation during manufacturing or transportation could affect their functioning. Irradiating the chips wouldn't make them radioactive but could reduce their long-term reliability.
The company had specs for the issue but had heretofore not needed to enforce these specs. In this case, however, Intel had to work with key suppliers to ensure that silicon and chips weren't exposed to radiation at any stage. In addition to attempting to cope with the existing crisis, Intel planned for an expansion of its crisis response if the evacuation zone grew and encompassed more suppliers.
A Nuclear Powerless Country
At the time of the quake, Fukushima Daiichi was supplying less than 1 percent of Japan's electricity needs. Other reactors in the quake zone - totaling 4 percent of Japan's power needs - were shut down for inspection. Yet quake- and tsunami-related damage to nonnuclear power plants and to Japan's electric grid, coming on top of Japan's unique split grid system, created power shortages in the eastern half of the country. Power outages and rolling blackouts became a problem for almost a month.
The government began an intensive media campaign called set-suden (Japanese for “power saving”). It encouraged citizens and companies to conserve as much power as possible by turning off lights, adjusting thermostats, and wearing clothing appropriate for the temperature. Retailers turned off neon lights, office buildings went dark, escalators stopped, trains slowed, and some manufacturers adopted staggered weekly schedules to reduce the weekday peak in demand. Rather than promoting consumption, the glowing giant neon signs of the retailers in Tokyo's Ginza district went dark to promote conservation.
As the Fukushima crisis worsened, public opinion turned against Japan's energy strategy, and the risks from dependence on foreign fossil fuels seemed the lesser of two evils. The extreme loss of confidence in nuclear power caused the government to progressively shut down all the country's reactors. By August 2011, three-quarters of Japan's nuclear reactors were shut down. By August 2012, only two of Japan's 54 reactors remained online. This created a more sizable disruption to the country's power supplies, reducing capacity by over 30 percent. More than three years after the disaster, power shortages still threatened Japan, especially during the hot summer months when electrical demand spikes.
When the Chips Are down
When the quake occurred, Intel's well-honed and extensively practiced crisis management processes sprang into action. Business unit crisis management teams had kickoff meetings on the day of the quake. On Saturday, March 12, Intel activated its Corporate Emergency Operation Center (CEOC) to help coordinate the response at the highest level. Intel split its response to the Japan disaster into two parallel streams of activities, which is Intel's standard approach for such incidents.
Emergency Management: Stabilizing the Local Situation
First, Intel's Emergency Management team ensured the safety of Intel's people and facilities in the disaster zone. Intel deployed pre-designated local Emergency Response Teams, who are the first responders in a disaster, and local Emergency Operations Center (EOC) personnel to stabilize the situation and prevent further casualties. At the time of the quake, 300 Intel employees worked in downtown Tokyo and a similar number in Tsukuba, about 100 kilometers northeast of Tokyo.
Intel's office in downtown Tokyo survived intact, but the Tsukuba facility was another matter. Intel had no fabs in Japan, but its Tsukuba facility housed 300 personnel who worked on materials operations, quality assurance, information technology, e-business, and related functions. Although the building wasn't structurally damaged, the quake broke fire sprinkler pipes in the ceiling. Ten inches of water flooded the offices and shorted the building's electrical systems. The Tsukuba office was uninhabitable.
Finding a temporary location for the 300 Tsukuba workers would not be easy given the 1.2 million buildings that had been damaged in the quake. Many companies had been forced out of their offices by the quake, tsunami, and Fukushima evacuations. “We have an organization at Intel called CRESD (Corporate Real Estate and Site Development), and so they worked night and day to try to find alternative work space,” said Jim Holko, program manager of Intel's corporate emergency management. Even with its corporate resources, Intel couldn't find a site large enough for all 300 people, so CRESD had to split the workforce across two locations. As a result, Intel also had to set up Internet and collaboration tools across the two sites.
Meanwhile, the company used its large global construction arm - which builds Intel's fabs - to accelerate the repair of the Tsukuba facility. Intel flew structural engineers, electricians, plumbers, and other technicians to Japan to inspect the building, define the requirements for the repair job, and find local contractors to do the repairs.
Business Continuity: Ensuring Global Operations
The second stream of Intel's crisis response, Business Continuity (BC), focused on Intel's products and processes. BC had to make sure that all the raw materials flows, chip making, and customer-related activities didn't stop - or that they restarted as soon as possible. Whereas the Intel's Emergency Management team took care of the safety issues within a couple of weeks of the quake, the BC side took six months.
Intel's first step in BC was to determine the impact of the disaster on the business operations of the company and its suppliers. Because Intel had no factories in Japan, supplier issues were its main BC focus. Intel assessed the status of 365 materials. By March 15, four days after the disaster, Intel knew it had no major problems with its direct (or “Tier 1”) suppliers. At worst, a few Tier 1 suppliers had a few days of downtime, but nothing that was a threat to Intel's production schedules.
Tracing the status of deeper tier suppliers - suppliers to Intel's Tier 1 suppliers - took longer. By March 20, Intel knew that Tier 2 also had only minor problems, but Tier 3, Tier 4, and deeper tiers had more substantive problems. Intel identified 60 suppliers who had issues. Many of them were single-source specialty chemical manufacturers with unique capabilities. Making chips with layers only a few atoms thick depends on highly-specialized, exotic chemicals. In many cases, only one supplier (and sometimes even only one plant) in the world knew how to make the molecules “dance in just the right way,” as Intel's Jackie Sturm, vice president, technology and manufacturing and general manager of global sourcing and procurement, put it.
Even as Intel resolved many uncertainties about the quake's im- pact on suppliers, the company could not be certain that it had identified all the impacts. “Everything that was a risk for us in that earthquake, [it turned out] we knew about in the first 10 days, but we didn't know that fact on the 11th day,” said Jeff Selvala, Intel's director, assembly test global materials.
Intel continued to probe suppliers, looking for any additional problems. News of issues at other chip makers would make Intel ask more questions, continuously digging for additional hidden disruptions and risks. Although no new problems appeared after day 10, Selvala said that Intel continued to search for more problems for at least a month.
More than 50 percent of Intel's assembly and test materials suppliers had manufacturing locations in Japan. The number was even higher for the deeper tiers, due to the high concentration of specialty chemicals makers in Japan. In all, Intel realized that 75 percent of assembly/test materials were at risk. One of the more significant challenges was getting enough silicon, which was the base material used for almost all of Intel's chips at every plant around the world. Five minutes after the shaking had stopped, Sturm was on the phone from the Narita tarmac, calling Intel's fab materials people to check the status of the company's silicon suppliers.
Quakes and Crystals
In 2011, the Shin-Etsu Handotai (SEH) plant in Shirakawa Japan was producing 20 percent of the world's supply of 300-millimeter silicon - the large platter-like wafers used to make chips. The factory's delicate crystal pullers slowly grew boules of silicon - heavy crystal ingots 300 millimeters in diameter - that were sliced into 600,000 to 700,000 wafers per month.
To give the 250 kilograms of molten silicon time to coalesce into a perfect crystal, the equipment slowly lifts the nascent boules out of the yellow-hot pool of molten silicon over a period of more than a day. The quake's shaking jostled these quiet pools, disordered the crystal growth, and ruined the crystals. When the power died, the gestating boules, representing tens of millions of dollars of chips, froze into stillborn cold gray lumps.
SEH's first order of business was to ensure the safety of its employees, three of whom had been injured in the quake. Next, SEH needed to inspect its facilities for damage to ensure it could restart production as quickly as possible. But even as the company worked to inspect and begin repairs at the affected site, the area came under threat from the nuclear reactor crisis and radiation leakage from Fukushima Daiichi, only 80 kilometers away. Moreover, the Shirakawa plants got their power from the Fukushima nuclear plant. Although the area around the Shirakawa was never under government evacuation orders, some concerned area residents did leave on their own. Local fears were so strong that TEPCO was forced to agree to pay all children and pregnant women living in the area at the time of the crisis 200,000 yen (about $2,600 at the time) each, as compensation for their fears and added costs.
SEH also initiated a series of communications to the business world about the status of damage to the company's facilities and its efforts to recover. The day after the quake, SEH reported three facilities damaged by the quake, including the Shirakawa semiconductor plant. By March 15, SEH announced it had found damage to the production equipment at Shirakawa but did not know how long it might take to repair the facility. By March 17, SEH decided to shift as much wafer production as possible to other SEH facilities. Inspections and assessment took almost a month. By April 11, SEH was in the process of recovering inventory from Shirakawa to increase wafer shipments. Not until April 28 could SEH restart partial production. The Shirakawa plant was the last SEH facility to return to full production on July 1, more than three and half months after the quake.
The larger obstacles to SEH's recovery came from the aftereffects of the quake. Between the quake damage and Japan's decision to take its nuclear plants off line, the country faced severe power shortages and rolling blackouts. Whereas individuals and many businesses experienced power outages as an inconvenience and a loss of productivity, other businesses - especially those like SEH who were running delicate industrial processes - faced potential safety concerns and equipment damage if the power went out. “We are requesting the electric power companies to provide a stable supply of electric power because we have facilities and equipment that need to be operated continuously due to the safety reasons,” pleaded SEH in its March 22 report on the company's post-quake efforts.
Acquire, Search, and Prolong Existing Supplies
Where possible, Intel sought to maintain business continuity using existing suppliers and prequalified materials. Its efforts were focused on three work streams. The first was to quickly acquire materials from its normal portfolio of prequalified suppliers. For example, Intel knew it had to acquire more silicon to fill the gap created by the downed factories. The company's first step was to approach its major suppliers, asking them to increase Intel's allocation. Despite suppliers' best efforts, the global wafer industry did not have the spare production capacity to replace what was lost in the quake.
Intel's second tactic was to search and secure the supply chain's inventories of critical materials. All supply chains have inventories at every level, some of it by design and some resulting from inefficiencies. Companies maintain inventories as a hedge against demand fluctuations and to take advantage of economies of scale in manufacturing and shipping. Inventory levels depend on lead times, manufacturing technologies, demand, and process uncertainty (see the section titled “Views on the Risks” in this chapter). At the same time, lack of intra-company communications, incorrect incentive systems and other factors can mean that companies often have more inventories than optimal. “You do have some amount of time, because you have inventory, your supplier has inventory, their supplier has inventory, and so on. And so there's a natural buffer there that you have a period of time to resolve issues,” Selvala said.
Third, Intel also worked to prolong the life of constrained sup- plies by minimizing the quantity consumed by each step of the chip-making process. For example, in one case, Intel diluted a key chemical, qualified it for use, and used the alternative formulation for eight weeks. Another engineering group found a way to clean off and reuse test wafers that would have gone to the scrap pile. Moreover, the company pushed the tactic up the supply chain - working with Tier 3 suppliers to minimize consumption of critical Tier 4 supplies. By boosting supplies from second-source suppliers, finding as much material as possible and prolonging its use, Intel hoped to fill the gap until the affected suppliers could resume normal production.
The Backup Response: Replace Disrupted Supplies
In some cases, Intel couldn't find enough prequalified suppliers with inventory or capacity to meet Intel's demands while its Japanese suppliers recovered. This unfortunate fact was especially true for sole-source suppliers. The lack of prequalified suppliers had the potential to create a lag during which time Intel could face production interruptions. Needless to say, the entire reason for the business continuity effort was to avoid such disruptions.
The threat of a production disruption led Intel to look for alternative suppliers and alternative products. Under normal circumstances, Intel's fab managers would resist quick qualification of alternative chemicals and materials. But these were not ordinary times. Intel would need to buy previously unqualified materials and quickly qualify them to ensure they met Intel's high quality standards.
Engineers sought alternatives to constrained materials and used fast-track qualification processes - jumping the normal queue of engineering work - to get the replacement materials into use. Intel also gave purchasing managers wide freedom to buy large quantities of materials “just in case” and accelerate the usual materials and spending approvals processes. “We were out there using letters of intent, if that was sufficient, or placing purchase orders if that was necessary, or non-cancelable purchase orders. Whatever was necessary to secure supplies. And we were doing that in real time,” Selvala said.
In seeking alternatives to disrupted supplies, Intel made sure that the lead-time of the alternative was less than the recovery time of the original supplier. Intel's supplier contracts often include “have made” rights, whereby Intel can have a proprietary material made by another supplier if the original supplier cannot meet the contract terms. Often, however, the lead-time on getting a material made elsewhere was longer than the anticipated recovery time of the original qualified sole-source supplier.
Collaboration for Better Crisis Response
Intel wasn't the only chip maker affected by the quake. The loss of the SEH plant created a global shortage of silicon. Other chip makers started calling Intel for help in locating silicon. Because the silicon shortage created imbalances in the PC supply chain, Intel assisted some of them in locating additional supplies. “If we were aware of capacity, we certainly tried to assist because the whole industry was down,” Intel's Jackie Sturm said. Even if Intel could make its chips, PC makers would not buy them unless they could get all the other chips and components from other suppliers so that they have everything needed to build complete PCs.
Intel also tried to help in other ways. It joined a group of Japanese companies in asking METI - Japan's Ministry of Economy, Trade, and Industry - to expedite the repair of the electrical grid around key suppliers and to exempt certain key facilities from the mandatory daily blackouts. Although it took some convincing, METI did help. Key suppliers were able to get continuous supplies of power even as blackouts affected other parts of Japan.
“We'll work with governments. We will work with competitors if that's what it takes. We'll work with our local authorities and try to engage wherever we think there's opportunity to help fix the situation,” Sturm said. Nor was Intel unique in this respect. In the other disruptions described later in this book, companies often collaborated with government, suppliers, and competitors.
Returning to Normal Operations: Winding Down Response
Some aspects of Intel's response were settled relatively soon after the quake. As the BC effort progressed, the frequency of meetings declined. During the first two weeks of the crisis, the crisis management teams of the Worldwide Materials Group had daily meetings over the status of issues such as silicon, chemicals, and back-end supplies. Then, they reduced the frequency to three times a week during April and May. In June, they further reduced the frequency to just once a week. At no time were factories down for lack of silicon, noted Sturm.
Once the recovery effort was under control, the CEOC closed on April 6. Similarly, the logistics crisis management team wound down on April 7, after figuring out the shipping lane issues and how to divert products to avoid radiation. Other business unit crisis management teams kept working until June 30. Overall, the business continuity effort took six months. Some follow-on actions continued thereafter, but operations were mostly back to normal. Complete rehabilitation of the Tsukuba office took 10 months. Throughout the entire crisis, Intel never had to halt production at any of its fabs.
The Globalization of Supply Chains
To understand why the Japanese quake and tsunami had global effects and how other kinds of distant disruptions can affect companies and economies, it helps to understand something about supply chains. From the point of view of a single company, a supply chain - which is actually a network of suppliers, sub-suppliers, and service providers - can be thought of as having five different aspects:
1.) The parts that go into the company's products,
2.) The identities of the network of suppliers who make those parts,
3.) The locations where parts and products are made, assembled, and distributed,
4.) The flows of parts and products (including the transportation links that move materials along the chain), as well as the flow of information and cash, and
5.) The inventories of materials, parts, and finished goods stored or being handled in various stages of the chain.
Each of these five aspects provides different insights into the risks to which supply chain operations are exposed.
Exploding the BOM: The “What” of Supply Chains
The first aspect of a supply chain for a given product, such as an automobile, encompasses all the materials and parts that go into that product - the “what.” To manage the myriad subassemblies, parts, and raw materials required to build a particular unit (a car, for instance, may have 50,000 parts), companies create a bill of materials (BOM) that lists the quantities of subassemblies, parts, and raw materials required to make one unit of a product. For example, the BOM for an automobile would include: one body, one engine, one transmission, four door assemblies, two axles, four brake assemblies, five wheel assemblies, one navigation system, and so forth. Each of these parts, in turn, includes other parts and subassemblies, and so on.
The assembled engine might include one engine block, six pistons, six fuel injectors, six spark plugs, twelve valves, etc. Each piston might include: one connecting rod, three piston rings, and so on. The BOM is a hierarchy that, if drawn, looks like a tree in which a set of leaves makes a twig, a set of twigs makes a branch, and the entire collection of branches makes the finished product.
Companies with multiple products will have a different BOM for each product, and each product will differ in the types and quantities of parts that go into that product. To make some planned number of products on an assembly line, companies use material requirements planning (MRP) software to ensure that they make or purchase the right quantities of every part on the BOM with sufficient lead time.
Given a production schedule, the planned number of units of each product during each time period multiplied by the number of parts per product to be manufactured during this time tells the company the number of parts to make or buy (e.g., one car with six pistons and three piston rings per piston needs 18 piston rings) for the manufacturing plan during that time unit. MRP takes a production schedule and generates a purchasing schedule, given the lead time for each part. Each supplier, in turn, uses its own MRP process to ensure that it, too, buys or makes the required raw materials and parts in time to deliver the requested parts to the product manufacturer by the scheduled delivery date.
Organizations in the Supply Chain: The “Who” of Supply Chains
The second aspect of the supply chain is of a network of facilities and companies that manufacture the parts of the BOM, assemble parts into finished goods, and then distribute and sell the products - the “who.” This aspect encompasses a spectrum of manufacturing strategies. At one extreme, a supply chain might be vertically integrated. In this case, a single company owns almost all the stages of production, in one or more facilities. For example, Ford's famous River Rouge plant was renowned for taking raw iron ore, glass, and rubber in on one side and rolling vehicles out of the other side of the massive, sprawling facility. A more modern example is Samsung, which makes many of the parts - such as processors, memory chips, and displays - for its own televisions, smartphones, and computer products.
At the other extreme is an outsourced supply chain in which a company buys complex, preassembled parts from a wide, tiered network of independent suppliers, with each supplier responsible for one or more steps in the production process, which might encompass a single simple part or a complete subassembly. In fact, some companies outsource their entire manufacturing operations, buying finished goods in retail packaging from contract manufacturers. For example, Cisco, Microsoft, and Apple do not have any manufacturing facilities of their own - they only handle the design, marketing, sales, and supply chain of their products.
Regardless of a company's level of vertical integration, the direct suppliers of the company are known as Tier 1 suppliers. Tier 1 suppliers would include the providers of steel or aluminum sheets and coil to Ford's stamping plants or contract manufacturers such as Flextronics International Ltd., Hon Hai/Foxconn, or Pegatron Corp, that manufacture computers, phones and tablets for Apple. A company's Tier 2 suppliers are the suppliers to the company's Tier 1 suppliers. Tier 3 supplies Tier 2, and so on. These echelons of tiers often correspond to the echelons of branches in the BOM tree.
Today's supply chains can be quite deep. For example, Intel traced tantalum, a metal essential its microprocessors, through as many as a dozen supply chain tiers back through the makers of tantalum-containing electronic components to the metal processors, smelters, ore exporters, ore transporters, ore consolidators, and then to the artisanal miners. In some cases, the structure of the BOM is more complicated as companies both buy from and sell to the same company, or where one part may “visit” the same supplier more than once for different manufacturing processes, a situation that is common in the information technology industry.
Manufacturing and Services Locations: The “Where” of Supply Chains Whether vertically integrated or outsourced, supply chains can also vary by their geographic deployment - the “where.” Manufacturers may choose to locate production facilities close to raw materials supplies (e.g., a chemical plant near an oil field), close to sources of labor (e.g., low-cost or high-skill regions), close to centers of demand (e.g., major customers or major population centers), in some industrial cluster location (close to other, similar manufacturers) or in a location influenced by government (e.g., via incentives and regulations).
The structure of the supply chain for acquiring and moving raw material and parts to final assembly (the so called “upstream supply chain”) is determined by the choice of suppliers. At most manufacturing companies, the choice of suppliers is managed by the procurement organization. Naturally, engineering, finance, and logistics also contribute to the decisions in order to ensure quality, capacity, financial viability, and timely deliveries of parts and raw materials. Lastly, departments such as risk management, compliance, and corporate social responsibility also influence supplier choice and factory location decisions.
To get the product to market (the “downstream” part of the process), the supply chain encompasses the distribution function. Distribution determines the location and operations of the company's warehouses and distribution centers. Distribution also usually manages the movement of the finished products to customers - be they retail distribution centers, retail outlets, e-commerce fulfillment centers, or directly to consumers. Many companies outsource distribution, too, either by selling their finished goods to wholesalers who distribute the product, or by using logistics service providers to handle warehousing and distribution to their down- stream echelons of retailers and consumers.
Going with the Flows: “How Things Move” in Supply Chains
All of these locations of supply, production, distribution, and demand are connected by flows - the fourth aspect of the supply chain. Supply chains encompass three essential types of flows: material, information, and money. The most salient and costly flows of a supply chain are the material flows. In general, materials flow downstream from mines and farms to factories that process raw materials, to the factories that make parts and subassemblies, to original equipment manufacturers (OEMs) that make finished goods products, to distributors and to retailers, and, finally, to end consumers. At each stage, companies add value to the materials, often differentiating them into many types of parts or products. Materials, parts, and products can travel on a variety of conveyances such as trucks, railroads, ocean freighters, canal barges, aircraft, and pipelines - the “how and where things move” in the sup ply chain. Intel, for example, manages 14,000 origin-destination “lanes” around the world, connecting the chipmaker to its suppliers, its network of internal facilities, and its customers.
At the same time that materials flow down the chain, money flows up the chain when consumers pay the retailer, the retailer pays the distributor, and so on. Information - in the form of, for example, forecasts, purchase orders, shipping notices, and invoices - flows in both directions to coordinate activities throughout the supply chain. In fact, both materials and money also go in both directions to some extent, as returns and defective goods travel back to the manufacturer, and as rebates and discounts flow from suppliers to customers. An increasingly important part of supply chain management involves the returns part of the supply chain, be it for responsible disposal, recycling, remanufacturing, or the return of packaging material.
Transportation carriers have their own operating strategies for how they handle and route freight. The two basic strategies are known as “direct operations” (DO) and “consolidated operations” (CO). In direct operations, a dedicated conveyance carries the cargo directly from origin to destination (like a taxicab). This is the case with full truckload movements, unit trains, and leased conveyances in all modes of transportation. Direct operations are not cost efficient for small shipments, however, so smaller shipments are usually consolidated geographically through transshipment hubs such as UPS's Louisville, FedEx's Memphis, or DHL's Leipzig terminal. Examples of CO modes (think public transit or passenger airline hubs) include less-than-truckload (LTL or “groupage”), boxcar trains, ocean container ships, and many others. CO also include in-vehicle consolidation, such as pickup and delivery operations in which shipments destined for a variety of places are loaded on a single vehicle for distribution (like postal service mail delivery).
Numerous factors - the economies of scale in conveyance size, the economies of scope underlying carriers' networks, the efficiency of handling modal transshipments, delivery time requirements, and the need to mediate between concentrated sources of supply (e.g., a large factory) and diffuse regional demand (e.g., a network of retail outlets) - all affect the choice of transportation mode in each specific situation.
The Story of Inventory: “Where Things Sit” in Supply Chains
Both the economics of production and the economics of transportation mean that products are typically shipped in batches of some minimum quantity. The economic order quantity is the production batch size (or shipment size) that has been optimized vis-à-vis inventory carrying costs and ordering costs. The batching of production and transportation implies that both manufacturer and customer must hold inventory - the manufacturer will hold finished goods inventory because of the efficiency of batch manufacturing and/or until it has produced enough for a cost-effective shipment; the customer company will hold this cycle inventory until it is sold off. In addition, each echelon in the supply chain may hold additional inventory - safety stock - to cover random fluctuations in customer demand or in parts' supply. Finally, because processes along the supply chain - especially transportation - take time, inventory also sits in trucks, on the high seas, or while undergoing some manufacturing process and is called the work-in-process (WIP) inventory.
Inventory is costly. It consumes capital on a company's balance sheet, consumes space for storage, and requires labor to put away, maintain, service, manage, and pick-and-pack. Inventory can de- grade over time (e.g., perishable food, medicines, and chemicals) or go obsolete (e.g., last-year's skirts or silicon chips). Consequently, companies try to minimize the amount of inventory they hold. In the 1950s and 1960s, Toyota pioneered the just-in-time (JIT) manufacturing and supply chain inventory management method as part of the Toyota Production System. The system was designed to reduce inventory along the supply chain while increasing product quality and service levels. This practice spread within Japan and then throughout the world. JIT and the related strategy of lean manufacturing/lean supply chain enable companies to operate with less inventory and be more responsive to changing market conditions.
Views on the Risks
Each of these five aspects of the supply chain offers respective in- sights into the many different risks in supply chains. The “parts” aspect of the supply chain is associated with materials availability, defective parts, and pricing risks associated with the various inputs to the final product or assembly.
The “who” and the “where” of the supply chain affect many geographic and operational risks such as natural disasters, supplier bankruptcies, and regulatory risks. Outsourcing - especially off-shore - reduces a company's level of control over the outsourced steps of the manufacturing process and can expose the company to geographic, legal/regulatory, and political upheaval risks. The “who” and “where” aspects also determine the natural resources footprints (e.g., energy, water, and carbon) and potential social responsibility risks in the supply chain.
The “flow” aspect of the supply chain depicts the range of connective risks in the logistics, financial, or information infrastructure that underlies the fabric of the chain. The material flow aspect includes risks in the timeliness of shipments and potential disruptions in key transportation terminals, lanes, and hubs. The information flow aspect highlights the vulnerability of global supply chains to information technology disruptions (e.g., computer failures, software glitches, or cyber-attacks). The money flow aspect depicts the vulnerability of commerce to financial crises, bankruptcies, and exchange rate risks. Finally, the “inventory” aspect of the supply chain includes geographic risks, product quality risks, and parts obsolescence. Yet, inventory also represents an opportunity to buffer many supply risks by allowing companies to maintain the flow of parts and products during a disruption.
Rising Vulnerability on all Sides of the Supply Chain
Intel's vulnerability to the Japanese earthquake, and the many examples in the rest of this book, arise from a series of trends that have pushed companies toward more complex, broader, longer, and more fragile supply chains.
More Trade, More Distance, Longer Lead Times, More Players
The leading driver of this growing vulnerability is the explosion of global trade. Global merchandise exports surged from $7.38 trillion in 2003 to $17.93 trillion in 2012. Rapidly declining costs of communications and growing efficiency of logistics are enabling all this trade, with the resulting lengthening of supply chains. Digital communications mean companies can more readily work with facilities, suppliers, and distribution centers on the other side of the world.
Containerization and larger conveyance sizes aid global trade by reducing transportation costs. In 1999, the largest container ships carried 8,700 TEU (twenty-foot equivalent unit) although 90 percent of container ships were 4,500 TEU or smaller (in order to fit through the Panama Canal, known as “Panamax” vessels). With the growth of trade came demand for bigger ships and the efficiencies they bring. By 2013, the largest ships carried 18,270 TEU and nearly 50 percent of the total fleet capacity was in post-Panamax ships. Larger ships, larger airplanes, longer trains, and larger trucks are all more efficient per ton-mile than smaller vehicles. Indeed, the trend in the industry has been to increase conveyance size in every mode of transportation, reducing the cost of long-distance trade in products. With the increase in size, however, comes a greater concentration of risk.
Global competition motivated companies to hunt for the best price and performance in global markets. As companies outsourced their manufacturing operations to distant lands and distant suppliers, lead time from order to delivery lengthened, meaning that there was more opportunity for things to go wrong. More actors were involved - from suppliers to service providers to multiple governments and regulatory regimes - thereby further increasing complexity and the probability of failure.
More Variety
Global trade, global competition, and the need for differentiation in the marketplace mean that companies now sell more varieties of each product - what companies refer to as SKUs (stock keeping units). For example, Colgate - one of the more than 16 competitors selling toothpaste in the United States - advertises 14 types of toothpaste. Higher SKU counts mean that each variant sells in relatively small quantity, making the problem of predicting demand difficult. The reason is that product demand is often subject to random variations.
The relevant measure of the variability of demand is the coefficient of variation: the ratio of the standard deviation to the mean. As the average sales of each SKU grow smaller, this ratio increases (owing to a linear decrease in the mean but only a square-root decrease in the standard deviation), resulting in more difficulties in forecasting demand, and leading to overstock/under- stock and higher costs. The first rule of forecasting - that forecasts are always wrong - is truer today than ever before.
More Technology, More Complexity
Many products have become more complex through the addition of embedded information and communications technology. Automobiles now contain between 30 and 100 microprocessors, with each subsystem of the car having its own controller and software. Every headlight, airbag, rearview mirror, seat, and door has its own dedicated microprocessor. Even simple products such as coffee makers and other home appliances use microprocessors and associated electronic systems. And new technology includes more than just electronics; products now rely on a growing variety of engineered materials, additives, pigments, and treatments that enable high efficiencies, performance, and market acceptance.
With product complexity comes the need to use more suppliers, who, in turn, may use more suppliers, leading to more complex supply chains. A car seat used to be like a piece of furniture and depended only on suppliers of cloth, leather, stuffing, and some metal or plastic framing. But modern car seats are technological gizmos that also include switches, motors, heating elements, sensors, and the ubiquitous microprocessor to control them. Even the seat materials themselves are more advanced, with high-tech foams and more durable, fashionable, and sustainable textiles.
Complex, Broad, Long, and … Vulnerable
Computers may make complex global supply chains more efficient and they work constantly to make them easier to manage, but they don't make them less risky. Companies can more readily manufacture complex products using complex supply chains, but the systems are inherently more fragile precisely because modern computers and communications enable tighter coordination and lean, inventory-less operations.
While such controls and processes make a company more competitive in normal times, they also make it more fragile to any event that disrupts the finely tuned global network of business machinery. In addition, complex supply chains mean “deep” bills-of-materials and thus many tiers in the supply chain. While companies may be able to pressure their direct suppliers to help manage risks, companies have little knowledge of these deep-tier suppliers and, in most cases, almost no influence over them to demand more resilience or adherence to a code-of-conduct.
In the end, the rise of global trade means that companies have more moving pieces stretched over greater distances and with less slack in the system. And with a growing global population and a growing global economy, significant supply chain disruptions are inevitable. Thus, when a quake hits Japan or anywhere else unexpectedly, the companies of the global economy find themselves shaking, too.
Yossi Sheffi is Elisha Gray II Professor of Engineering Systems at MIT and Director of the MIT Center for Transportation and Logistics. He has worked with leading manufacturers and logistics service providers around the world on operations and strategy issues and is an active entrepreneur, having founded or cofounded five successful companies. He is the author of The Resilient Enterprise: Overcoming Vulnerability for Competitive Advantage and Logistics Clusters: Delivering Value and Driving Growth, both published by the MIT Press.
SC
MR

Latest Supply Chain News
Latest Podcast
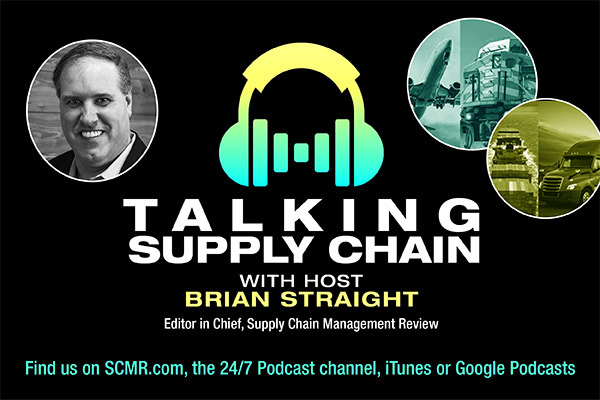
Explore
Topics
Latest Supply Chain News
- Strengthening customer fulfillment: Building a strategic stakeholder network
- The hard job of teaching soft skills
- Trump picks former Wisconsin congressman Sean Duffy for DOT secretary
- Made in Mexico, manufactured by China
- Retail sales see gains in October, reports Commerce and NRF
- Balancing green and speed: Home delivery insights from the pandemic era
- More latest news
Latest Resources
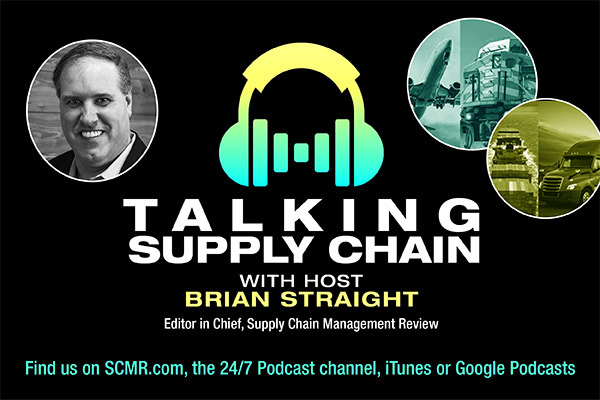
Subscribe

Supply Chain Management Review delivers the best industry content.
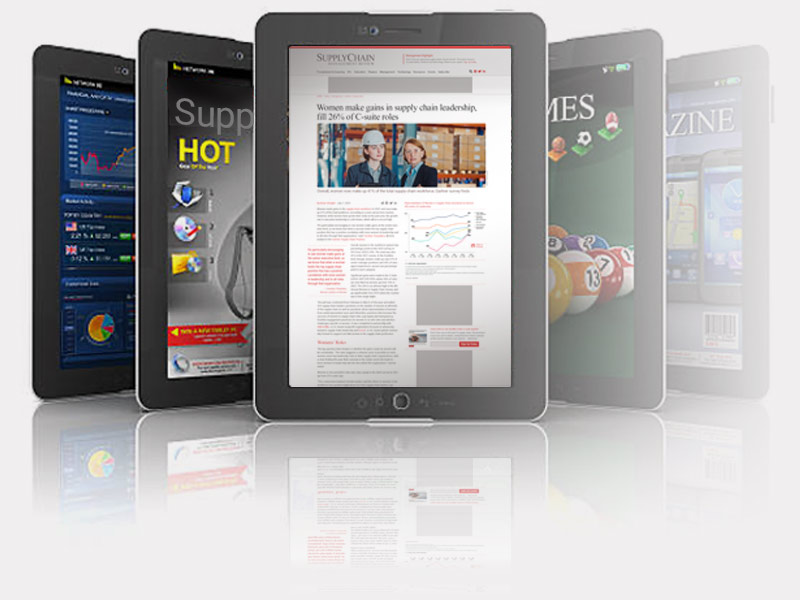
Editors’ Picks

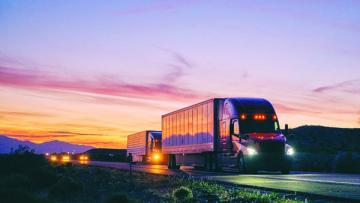

